Algerian Journal of Environmental Science and Technology is an international, peer-reviewed research journal which publishes original research contributions to scientific knowledge to promote the theory and practice of environmental science and technology, innovation, engineering and management.
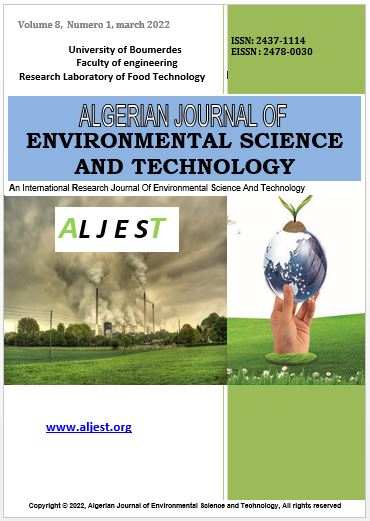
Vol 9, No 4 (2023)
Table of Contents
Articles
M. Amrani, D. Benazzouz
|
|
A. Bitam-Derridj, Z. Adjerid, K. Ouzegane
|
|
M. Laraba, M. Derradji
|
|
A. Hattali, O. Bouras, S. Hanini
|
|
M. Naili, D. Telaidjia, F. Eddaoudi
|
|
A. Benahmed, N. Benbrahim, F. Debab, Z. Zizi
|
|
M. Metaiche, J. Sanchez, A. Sirat, C. Charmette, B. Sala
|
|
S. Cheddad, A. Haouchine
|
|
A. Ouamrouche, K. Benyounes, A. Benmounah
|
|
K. Bourenane, ABD. Haddar, A. Haddar, I. Zerouki, D. Abdessemed
|
|
H. Bouzemlal, M. Boumahdi, S. Hanini, M. Laidi, A. Ibrir, M. Roubehie Fissa, N. Boucherit
|
|
I. Bioud, A. Semar, A. Laribi, S. Douaibia, M.N. Chabaca
|
|